
© Photo credit: NASA
A TALE OF TWO BLACK HOLES
Information provided by Marco Cavaglia, Professor of Physics at the Missouri University of Science and Technology.
As you are reading these words, a catastrophe is about to happen.
Somewhere in the deep recesses of the Universe two black holes are swirling in space at almost the speed of light. In a few seconds they will collide and merge. Most of their energy will be trapped in a region of space from which there is no way out. However, some of it will escape the Armageddon, causing space to deform and time to warp over a cosmic expanse of billions of light years. As amazing as all this may sound, perhaps the most incredible aspect of this story is how we came to understand their physics and how we built instruments capable of detecting these cosmic collisions. Black holes are no match, even for human ingenuity!
Like many stories of human ingenuity, the tale of these black holes begins in a time of tragedy. While in 1915 many were coping with the madness of World War I, north of the Alps, two German physicists were working the math of what, 50 years later, would become known as black holes. Albert Einstein had just published his Theory of General Relativity telling the world that space and time can be molded like rubber. Gravity is not a force, but the effect of the curvature of “space-time.”
Karl Schwarzschild, an army lieutenant on the eastern front battling both enemy gunfire and the disease which ultimately killed him, read about Einstein’s theory and set his math skills in action. Before his early death just a few months later, he gifted the world with the first exact solution of the equations of General
Relativity.
Unbeknownst to both, the Schwarzschild solution would spur decades of theoretical studies and astrophysical observations leading to one of the most astounding discoveries in science—black holes.
What is a black hole?
A black hole is a region of space where gravity is so strong that nothing—not even light—can escape from it. We call this region of space “trapped.” The trapped region is separated from the rest of the universe by a surface called the event horizon. The event horizon of a non-rotating black hole has a round shape, like a sphere. Just like the Earth has a gravitational pull and mass, so does a black hole.
Think of what might happen if we could take the Earth and compress it into the size of a marble to concentrate its mass and gravitational pull. Concentrate that pull enough so not even light can escape, and you have a black hole.
The size of the hole is measured in terms of its Schwarzschild radius—the larger its mass, the larger its radius. For a black hole with the mass of the Sun, the Schwarzschild radius is approximately 2 miles. That means if we could compress the four nonillion pounds of hydrogen and helium that make up the Sun in a region of space 2 miles wide, that would create a black hole.
Can you travel into a black hole? What would happen if you did?
Once an object crosses the event horizon of a black hole, there is no way back. The gravitational field of the black hole warps space-time so strongly that an infinite amount of energy would be required to overcome its pull. One can imagine a black hole as a “waterfall of space-time.” No matter how fast you try to swim outward, the inflow of gravity pulls you back. So, if you were to encounter a black hole, would you be doomed? Not so fast! It depends how close you get.
A common misconception of black holes is that they suck in everything around them. If we replaced the Sun with a black hole of equal mass, the Earth would continue on its orbit as if nothing had happened. However, the closer you get to the event horizon, the more difficult it is to break free from its gravitational pull.
Even if it is theoretically possible to get close to a black hole and come back to tell about it, it may not be a good idea to do so. If you were to dive headfirst into one of these cosmic monsters, the black hole would pull on your head more than on your feet. This could lead to what physicists call “spaghettification”. If a black hole with the mass of the Sun were located at the Science Center, a person located 100 miles away in Rolla would experience a pull between their head and feet greater than 10,000 times the force due to Earth’s gravity. That would certainly be very uncomfortable!
Although it may seem counterintuitive, the larger a black hole is, the flatter its surface, and the weaker its local gravity. Supermassive black holes, weighing millions of times our Sun, like those residing at the center of many galaxies, would not cause the same degree of spaghettification as smaller black holes. In other words, “small” black holes could turn unsuspecting guests into spaghetti, but supermassive black holes would not.
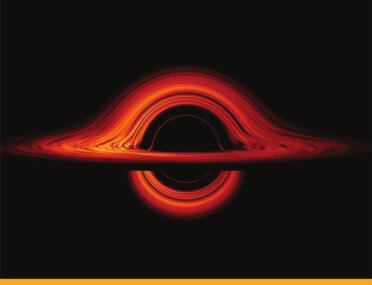
What would we experience when passing through the event horizon of a super-massive black hole?
Absolutely nothing! The surface of a black hole is just like empty space. Only if we tried to do a U-turn, we would realize that something is wrong as we would inexorably continue to fall toward the black hole center. Inside a Schwarzschild black hole time becomes space and space becomes time. Since time cannot be made to go backwards, the travel direction cannot be inverted. Since no information can be received from the interior of a black hole, we have no way to know what lies inside. Moreover, the equations of General Relativity break down at the center of the hole. It is likely that General Relativity must be extended or modified, but after 100 years we still don’t know how.
While we cannot say for certain what lies inside black holes, General Relativity and astronomical observations show us that happens on the outside. The closer to a black hole, the slower time flows as seen from far away. Therefore, nothing can be seen crossing the event horizon by an outside observer. However, any matter present around the black hole heats up as it falls onto the event horizon. This allows astronomers to infer he presence of the black hole through the emission of highly energetic light, such as X-rays.
Two years ago, the Event Horizon Telescope produced the first ever image of the “shadow” of a supermassive black hole located at the center of M87, a galaxy located about 50 million light years from Earth, on the surrounding accretion disk.
Observing the shadow of an object is not the same as observing the object itself. To be sure that what we observe is really a black hole we must prove an event horizon is present. Black holes are objects made of gravity, so in order to “see” the event horizon we have to use gravity rather than light. General Relativity tells us that if we bump a black hole, it will emit gravitational waves. Much like a struck bell stirs the air around it causing sound waves, an “out-of-shape” black hole stirs its surrounding space-time producing gravitational waves.
These “ripples of space-time” can be observed with very sophisticated detectors, called laser interferometers, that measure the space-time distortion caused by the waves as they pass through. Black holes produce very distinctive gravitational waves, unlike any other material object. If you can detect one of these waves, you know for certain that it must have been created by a black hole.
On September 14, 2015, a 1.3 billion-year-old space-time ripple, which began with an energy burst greater than the energy of the whole visible Universe, warped the space around Earth by a tiny fraction of the size of a proton. The two twin Laser Interferometer Gravitational-wave Observatory (LIGO) detectors in Louisiana and Washington state observed in unison this minuscule cosmic jolt. The signature of the wave which they recorded was unmistakably that of a collision of two stellar-mass black holes. This event provided the smoking gun that black holes exist as predicted by Einstein, and they can even merge.
One hundred years after their theoretical work, Einstein and Schwarzschild would have been elated by the discovery made by the thousands of physicists, technicians and engineers of the LIGO Scientific Collaboration and the European Virgo Collaboration who built, conceived and operated these amazing detectors through decades of painstaking work.
Five years and tens of binary black hole detections later, we have learned that black holes are ubiquitous in the Universe. They range in size from stellar sizes to supermassive objects as heavy as billions of suns. They collide, merge and grow. They form in catastrophic star collapses. Perhaps some of them came into existence in the very first moments of our Universe. We know that they are not eternal and may disappear in a very distant future in bursts of radiation. Like many other scientific discoveries that started in the shadows of devastating wars, the imagination of Einstein and Schwarzschild has set humanity on a path to knowledge that still continues today.
That is part of the beauty of science.

1
Black holes come in all sizes.
We only observed black holes with masses ranging from a few to several tens of billions of times the mass of the Sun. However, Einstein’s General Relativity tells us that black holes can be created with essentially any mass—from subatomic values to the mass of the whole universe.
2
Black holes have no “hair.”
No matter how black holes are formed, the only physical quantities that determine their properties are their mass, spin rate and charge. Like bald heads of the same size, complexion and age look all the same, so do black holes.
3
Black holes are not really black.
Due to the quantum nature of particles, black holes can evaporate by emitting radiation. In other words, they shine (in many shades of) “gray” rather than being pitch black.
4
Black holes can merge, but cannot split.
If you collide two black holes, you can form a bigger one. Astronomers have observed this process tens of times. However, the laws of black hole thermodynamics forbid the reverse process. (Or so we think…)
5
Black holes can ring.
Like a glass partially filled with water can vibrate at a specific frequency, so can a black hole with a given mass. The universe uses a “black hole” harp to play its symphony in gravitational waves.